Twin gene-editing system gives twice the efficiency
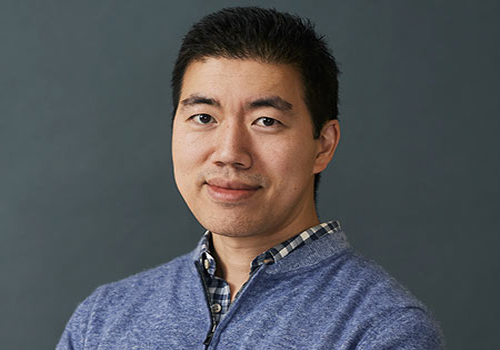
BY Yahya Chaudhry
Harvard Correspondent
A team of researchers led by Harvard and Broad Institute
scientists has developed twin prime editing, a new, CRISPR-based gene-editing
strategy that enables manipulation of gene-sized chunks of DNA in human cells
without cutting the DNA double helix.
Because it can make larger edits than previously possible,
the new technique could make it possible to study and treat genetic diseases
arising from loss of gene function or complex structural mutations, such as
hemophilia or Hunter syndrome.
In the paper, published in
the journal Nature Biotechnology, the researchers detail how they
developed twin prime editing (PE), which uses a prime editor protein and two
prime editing guide RNAs (pegRNAs) for the programmable replacement or excision
of DNA sequences at endogenous human genomic sites without requiring
double-stranded DNA cuts.
When combined with a site-specific recombinase, twin PE
allowed integration of gene-sized DNA of more than 5,000 base pairs into the
human genome at sites chosen by the researchers. Because large structural
variants are found in many human disease-causing alleles, this technique may
help treat genetic diseases. The study used twin PE plus recombinase to edit
genes linked to Hunter syndrome, phenylketonuria (PKU), Duchenne muscular
dystrophy, and hemophilia.
“A major remaining challenge in mammalian cell gene
editing is our inability to make targeted gene-sized insertions at sites of our
choosing,” said David Liu, the paper’s senior author, Thomas Dudley Cabot
Professor of the Natural Sciences, and a core faculty member of the Broad
Institute. “Such a capability could advance gene therapy by enabling genes to
be restored in their native sequence locations, without increased risk of
cancer from semi-random or uncontrolled integration at other locations in the
genome.”
This work was performed by members of Liu’s lab,
including former postdoctoral fellow Andrew Anzalone, former graduate student
Chris Podracky, postdoctoral fellow Xin (Daniel) Gao, and current graduate
student Andrew Nelson.
This new method is designed to overcome some of the
limitations of existing gene-editing techniques, and build on the strengths of
prime editing.
In 2016 the Liu group developed base editing, an
efficient and precise genome-editing method that functions like a genetic
pencil, chemically rewriting one DNA base to another without completely
breaking the DNA backbone. The Liu group developed two classes of base editors
that can correct four of the most common kinds of single-letter mutations, which
collectively account for about 30 percent of known disease-associated human
genetic errors. Three years later, the group developed prime editing, in which
a reverse transcriptase directly copies edited DNA sequences into a specified
target site from an extended guide RNA, again without requiring double-stranded
DNA breaks. Like a genetic word processor, prime editing lets researchers
search for one DNA segment and swap it for another.
Now, twin PE lets researchers search and replace larger
DNA segments, again without breaking double-stranded DNA.
“Using twin prime editing is like using two word
processors at the same time to simultaneously write different parts of the same
paragraph,” said Liu, who is also a Howard Hughes Medical Institute
Investigator. “In the process, you can create that paragraph more efficiently.”
The researchers developed twin PE by using simultaneous
prime edits to create both strands of the edited DNA. By itself, twin PE
enables efficient deletions, insertions, and substitutions of hundreds of base
pairs, achieving larger edits than simple prime editing. Seeking the ability to
make even larger edits of up to thousands of base pairs — a size large enough
to include many whole genes — the team installed Bxb1 recombinase “landing sites”
at targets site in human cells. The recombinase then mediated the insertion of
large DNA cargo sequences into the landing sites.
The team tested twin PE plus Bxb1 recombinase by
inserting large DNA into a variety of target sites in the human genome, including
at “safe harbor loci” thought to be ideal for gene therapy because inserting
genes there has been found to not induce cancer or other apparent toxicities.
The researchers also applied twin PE-mediated deletions
to target DMD. Pathogenic DMD alleles, which cause Duchenne muscular dystrophy,
commonly contain large deletions in exonic regions that result in frame-shifted
transcripts. These experiments show that twin PE can generate large deletions
at therapeutically relevant loci in humans with far fewer potentially harmful
byproducts than paired Cas9 nuclease strategies.
The team also used twin PE and a recombinase to install
a pathogenic 40,000 bp inversion that causes Hunter syndrome. Their success
suggests that the combination might eventually work as a therapeutic strategy
for correcting other large or complex pathogenic gene variants.
Going forward, Liu is optimistic that other
recombinases can work effectively with twin PE.
“We hope to apply twin PE to some basic science and
some therapeutic questions, while continuing to develop this strategy to
increase the efficiency of large gene size integrations,” Liu said.
Podracky, who joined the lab in 2015, said he hopes
that the twin PE plus recombinase technique will both be studied and be an
inspiration for scientists across the country.
“We could see other academic labs take the technology
and put it put it to good use,” Podracky said. “If this enables [scientists] to
realize their gene-editing ideas more readily, that would be a success.”
This study was
supported by the Merkin Institute of Transformative Technologies in
Healthcare, the National Institutes of Health, and the Howard Hughes
Medical Institute.